Updated 5 months ago
Perovskite solar cells: why they’re the future of solar power
Written by Ben Zientara Ben ZientaraBen Zientara is a writer, researcher, and solar policy analyst who has written about the residential solar industry, the electric grid, and state util...Learn more

Why you can trust SolarReviews
SolarReviews is the leading American website for solar panel reviews and solar panel installation companies. Our industry experts have a combined three decades of solar experience and maintain editorial independence for their reviews. No company can pay to alter the reviews or review scores shown on our site. Learn more about SolarReviews and how we make money.
At the leading edge of scientific discovery and renewable energy research, a class of materials called perovskites has excited the imaginations of some of the world’s top scientists and engineers.
These incredible materials have the ability to generate more electricity from the sun than almost anything else, potentially at a much lower cost than traditional silicon solar cells. But perovskites have so far required a lot of testing and trial-and-error, and no single application has reached the point of commercialization. The study of perovskite solar cells has come a long way in a very short time, but there are some big hurdles to overcome.
Because of the work of many dedicated researchers, some perovskite products may be coming to the market within the next year or two, so it’s important to learn about them now. Unfortunately, most of the information about perovskites on the web is directed at the researchers and scientists who study and work with these materials, and that stuff is necessarily pretty dense and technical.
What follows below is our attempt to cover perovskite materials in detail, but without a lot of the technical jargon and hard-to-understand concepts of a scholarly journal article. Someday soon, you may be able to have perovskite solar panels installed on your roof, so it’s time to learn about these exciting materials and what they might mean for rooftop solar in the very near future.
Key takeaways
Perovskites are materials with specific crystal structures that exhibit a photovoltaic (electricity from light) effect.
These materials have the potential to revolutionize the solar industry by greatly increasing efficiency and reducing the cost to manufacture solar panels.
Scientists have been working hard on perfecting these materials since 2009, and commercially-available solar cells may be coming out in the next year.
The advantages of perovskites for making solar cells are hard to overstate, but there are drawbacks—such as the presence of lead in these materials—that must be overcome before they can become truly widespread.
What is a perovskite?
Perovskites are a class of materials with a distinctive crystal structure similar to a mineral of the same name first discovered in Russia in 1839. Many varieties of perovskites exist, but the most interesting of these for the solar industry are crystals built out of organic and inorganic molecules connected to atoms of lead or tin.
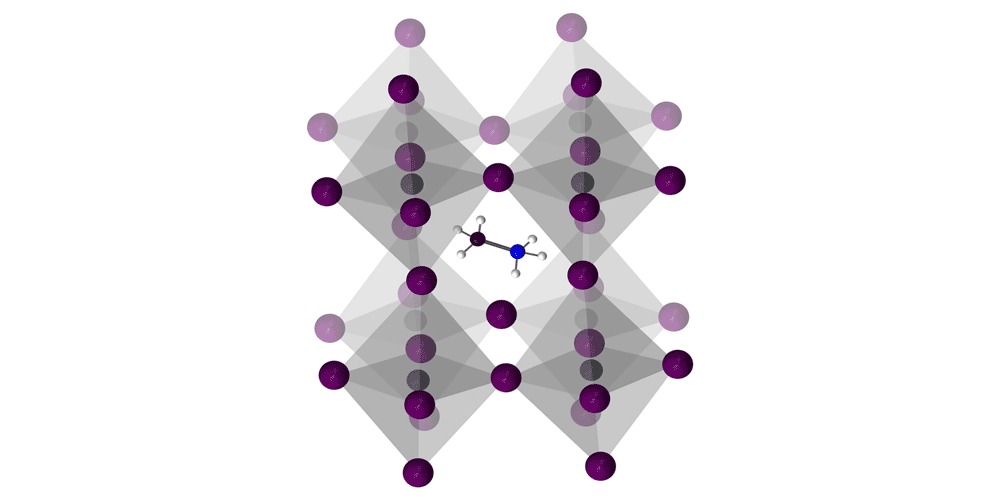
The structure of one perovskite material used in solar cells. Image source: Science Advances
The image above is a representation of the structure of one kind of lead halide perovskite crystal. It has a grid of 8-sided molecules called lead halides (an atom of lead connected to 6 halogen atoms of either iodine, chlorine, or bromine), surrounding a smaller molecule called a methylammonium cation (we promise this is as science-y as this article gets).
Why perovskites are important
Perovskites are exciting for several reasons, but the reason we’re going to talk about relates to the photovoltaic effect, which means “energy from light.”
The tin or lead present in these materials are good for making solar cells in the same way silicon is used for making solar cells. Atoms of these elements are ideal for forming molecules with other atoms that are semiconductor materials, whose electrons can be excited by light energy and directed along a wire to produce electricity.
Unlike silicon crystals, perovskite crystals are pretty easy to make under fairly ordinary conditions. Silicon must first be heated to extremely high temperatures to produce material with the right purity and crystal structure to make electricity; perovskites can be created by mixing chemicals in solution and coating a surface with that solution. The process is a bit more complicated than we’re letting on, but for the most part, producing the perovskite solar cells of the future will likely be significantly cheaper and easier than making silicon cells.
Perovskites are also important because their ability to make electricity can be “tuned” by controlling the kinds of molecules that are produced in the manufacturing process. This tuning results in materials with the ideal “bandgap,” which is the amount of energy needed to push an electron to a higher energy level so it can carry an electrical charge across a circuit.
Perovskites, efficiency, and the bandgap
Every atom in the universe has one or more electrons floating around its nucleus, and the negatively-charged electrons are attracted to the positively-charged nucleus. Molecules made up of many atoms form based on the number of electrons each atom has, and the shared electrons float around the molecule. The outermost electrons are said to be in the “valence band” of the atoms they orbit.
Solar electricity is generated when photons of light “bump” the outermost electrons of a semiconductor material to a higher energy state, thereby pushing them out of the valence band and into the “conduction band” of the molecule. The minimum amount of energy needed to push an electron from the valence band to the conduction band is called the bandgap.
When an electron is pushed into the conduction band, it is no longer stuck in the orbit of the molecule; instead, it becomes a charge carrier that can move through the material it’s part of, carrying electrical energy that we can use.
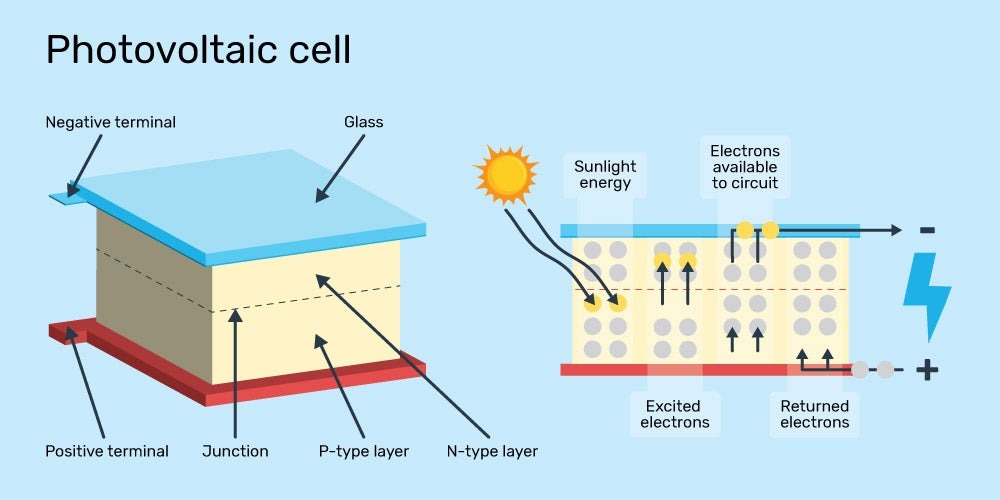
How sunlight causes electrons to become charge carriers in a solar cell.
Photons of different colors of light carry different amounts of energy, measured by units called “electronvolts” (eV). Photons of visible light have energies of between 1.75 eV (deep red) and 3.1 eV (violet). An ideal photovoltaic material has a bandgap of 1.34 eV, because that’s the point at which the maximum amount of visible light will convert electrons to charge carriers.
There’s a concept in solar related to the bandgap called Power Conversion Efficiency, or PCE, which is the amount of solar energy that can be converted to electricity by a solar cell. A solar cell that uses a single connection (more commonly called a junction) between layers of positive and negatively-charged materials with the ideal bandgap can convert 33.7% of all incoming light to electricity. This ideal efficiency is called the Shockley–Queisser limit, named after the physicists who discovered it.
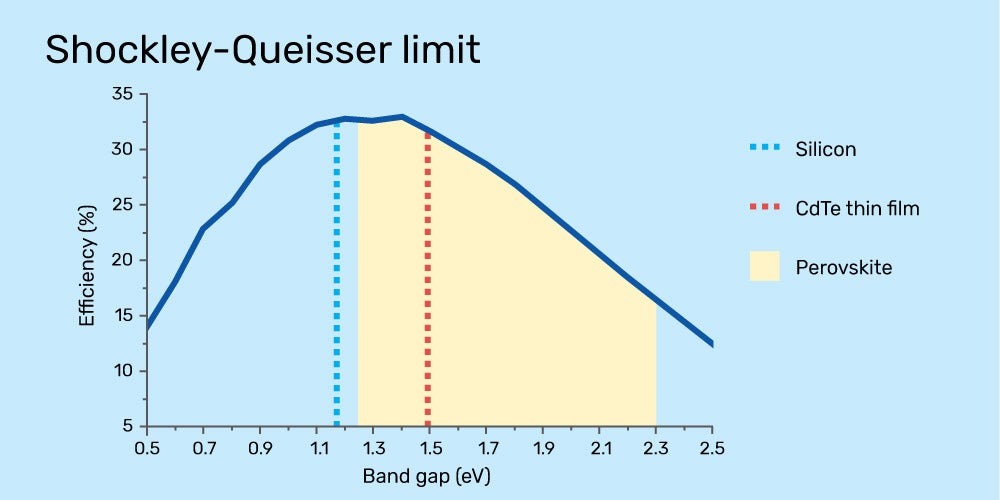
Perovskites can be tuned to various bandgaps within a wide range, while other materials only have one.
The trouble with the Shockley–Queisser limit is that no single material we know of has the perfect bandgap to reach it.
Silicon solar cells have a theoretical bandgap of about 1.2 eV, meaning they have a maximum PCE of around 32%. The best perovskite materials can reach about 31%, but there are reasons why it might be better to use a perovskite material with a higher or lower bandgap, even though it wouldn’t be ideal for use by itself. That brings us back to the concept of “tuning the bandgap,” as we discussed above.
By controlling the chemical makeup of a perovskite crystal, materials scientists can manufacture perovskite materials to have a bandgap very close to ideal for converting light to electricity, but they can also create multi-layered perovskite solar cells in which each layer has a different bandgap. Having multiple layers means high-energy photons excite electrons in layers with a wider bandgap, and low-energy photons excite electrons in layers with a narrower bandgap. In this way, more of the total solar energy gets converted into electricity.
This technology has shown great progress in recent years, and multi-junction cells made up of perovskite layers of varying bandgaps have already reached a conversion efficiency of 26%, despite having been researched since just 2013. Moreover, a tuned layer of perovskite can be added in a “tandem cell” arrangement with a traditional silicon cell to capture photons the silicon can’t convert, thereby increasing power conversion efficiency.
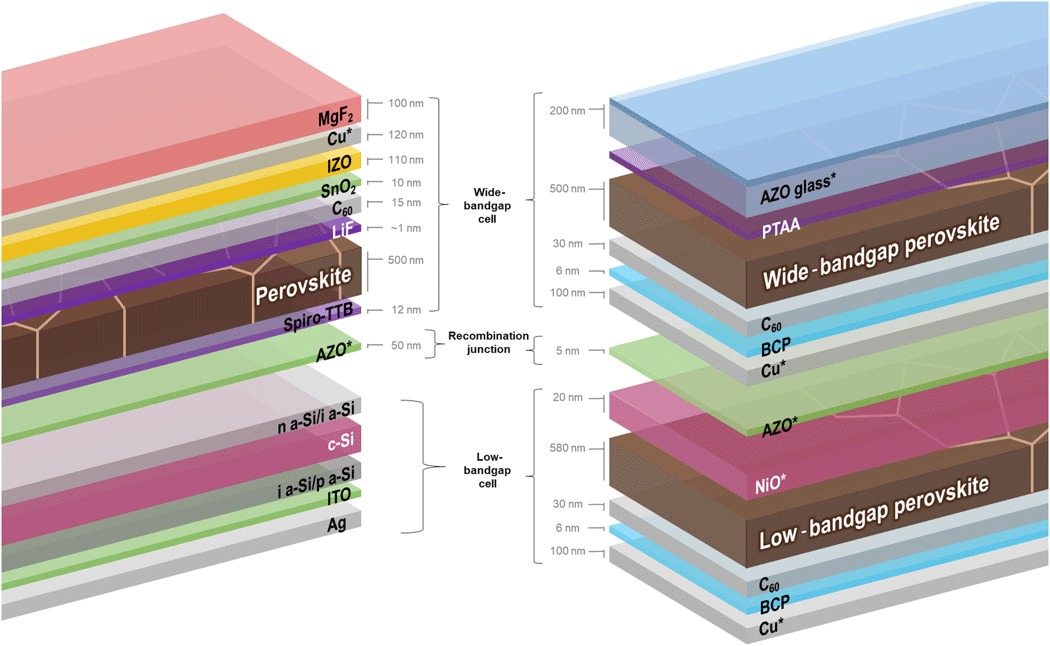
The structure of perovskite-silicon tandem solar cell (on the left) and perovskite-perovskite tandem solar cell (on the right). Image source: Science Advances
Some day, combining perovskite solar technology with the best of silicon-based tech might be the key to unlocking solar cells that can turn 50% of sunlight into electricity. That would be huge, considering that Maxeon currently has the highest efficiency rating on the market with their solar panels converting 22.8% of electricity into usable power.
The key takeaway of the above is this: perovskite cell efficiency might never be as good as the best silicon solar cells, but it will be good enough at a low-enough cost that the amount of electricity they produce per dollar spent on them will be much, much lower than silicon-based photovoltaic products.
The promise of perovskite solar cells can’t be understated. The reductions in cost they might provide is so exciting that the U.S. federal government has invested millions of dollars in perovskite research through the Office of Energy Efficiency and Renewable Energy. In 2020, $20 million in funding was available for perovskite research, spread among initiatives to develop cell technology, manufacturing best practices, and cell testing procedures.
How are perovskite solar cells made?
We’re going to keep this simple, because it isn’t necessary to know all the chemistry that goes into creating these things to understand how they work. Basically, perovskites can be created using “wet chemistry” in which materials like methylammonium lead iodide, methylammonium halide, and other additives are mixed together in a solution. The mixture is then deposited on a substrate like glass, metal oxide, flexible polymers, a silicon solar cell, or even transparent wood (wow!).
The deposition of the perovskite solution is usually done via spin-coating, which is kind of the same concept as the Spin-Art machines children use to make splotchy paintings on thick paper. The solution is dripped or sprayed onto the substrate, which is then spun at a high enough speed to spread a thin layer of the solution across its surface. When the solvents in the mixture evaporate, they leave behind perovskite films; thin layers of perovskite crystals ready to be wired into a solar cell.
Different types of perovskite solar cells
All solar cells, no matter what they’re made of, have certain things in common.
They must all have at least one negative layer and one positive layer of photovoltaic material; and they must have conductive front and back electrodes to carry the sun-charged electrons from the negative layer along a wire to produce electricity before returning them to the positive layer. Once mounted in a solar module, the cells are sealed in an encapsulation layer to protect them against damage from weather.
There are essentially two different types of perovskite solar cells: thin-film cells with perovskite as the only photovoltaic material, and tandem cells, which have either multiple layers of perovskite or a thin perovskite layer on top of traditional crystalline silicon.
To complicate matters a little, there are also thin-film tandem cells with a perovskite layer on top of copper indium gallium selenide (CIGS), which is an already-perfected thin-film solar technology.
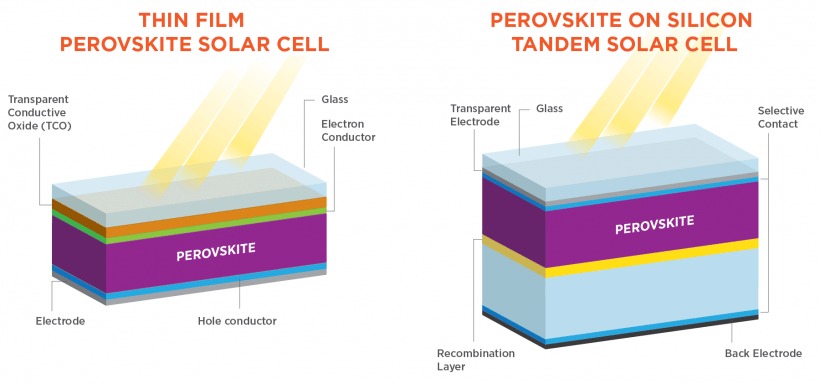
Thin-film vs tandem solar cell structure. Image source: U.S. DOE
Pros and cons of perovskites
As we discussed above, perovskites are exciting because they convert solar energy into electricity nearly as well as silicon, but can potentially be manufactured much more cheaply. Unfortunately, there are downsides to perovskites, as well.
Advantages
Relatively easy to manufacture and deposit onto a surface using low-cost processes
Potential for high power conversion efficiency
Tunable bandgap, meaning it can be manufactured to be almost ideal for solar energy generation
Production requires 20 times less material than silicon cells, and doesn’t use rare earth metals
Manufacturing process is much less energy intensive than that of traditional solar cells
Disadvantages
Perovskites break down over time when exposed to moisture, light, heat and oxygen, meaning there needs to be additional technologies developed to stabilize the cells for widespread use
The very best perovskites at generating energy contain lead, which is a neurotoxin; however, the industry is working on ways to reduce potential perovskite toxicity
Perovskite cells are not yet ready for commercial sales
The advantages of perovskite materials for photovoltaic applications are hard to overstate, and researchers have made some progress in solving the drawbacks of lead content and material stability.
Some possible solutions include replacing lead perovskites with tin-based ones (although experimental tin perovskites have much lower power conversion efficiency), and special polymer encapsulants that bind to the lead and stop it from leaching out in case the cells become damaged.
Who makes perovskite solar cells and when can people buy them?
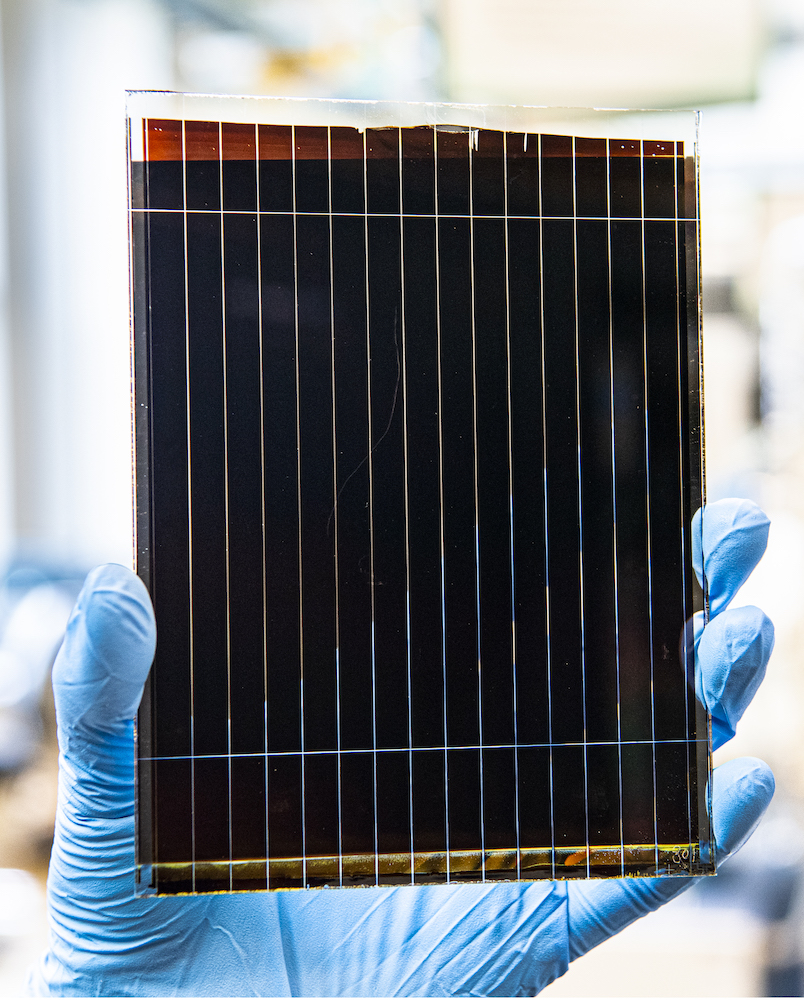
Perovskite solar cell in a lab. Photo: Dennis Schroeder / NREL
Nearly all perovskite solar cells are currently made by researchers in places like the National Renewable Energy Laboratory (NREL), to be poked and prodded and tested for their ability to make solar power and long-term stability and durability under common environmental conditions. These are mostly postage-stamp sized test cells, not ready for sale to the public. There are some companies, though, who say that large-scale commercialization of perovskites is not far away.
OxfordPV
One such company, Oxford PV, touts its high-efficiency perovskite/silicon tandem cells as being nearly ready. Oxford’s big breakthrough in perovskite photovoltaics was the announcement of a 29.52% efficient tandem cell in December, 2020—the highest efficiency ever verified in a solar cell at the time.
In 2021, Oxford released news that work on its manufacturing facility in Brandenburg, Germany was complete, and that production would begin sometime “in 2022.” But in announcing the completion of the facility, Oxford simultaneously broke ties with Meyer Burger, the partner that helped them build it. That parting-of-the-ways led to a rather messy separation, and as of this writing, the Brandenburg facility is not making tandem perovskite-silicon cells as expected.
Qcells
Perhaps the second likeliest candidate to market perovskite products is Qcells, which has said it is building a perovskite manufacturing line into a new South Korean facility. Qcells is one of the largest solar module manufacturers in the world, and it has plans to expand its facilities across the globe, including doubling the size of its facility in Dalton, Georgia, which is already the largest solar manufacturing plant in the western hemisphere.
Saule
Another company, Saule Technologies, is currently looking for licensing partners for its “kinetic solar blinds,” which feature inkjet-printed perovskites added to wide-bladed venetian-style blinds, as seen in the image below.
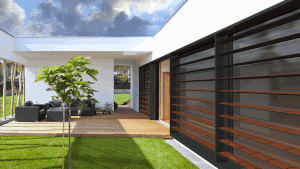
Image source: Saule Technologies
Saule Technologies is exciting because it was founded by Olga Malinkiewicz, who made breakthrough discoveries in perovskite technology while working as a PhD student at the University of Valencia. Her work was one of the catalysts for the explosive growth in perovskite research. Despite the promise of these products, it should be noted that Saule has been looking for a partner for this technology for nearly 2 years, without a taker.
Ben Zientara is a writer, researcher, and solar policy analyst who has written about the residential solar industry, the electric grid, and state utility policy since 2013. His early work included leading the team that produced the annual State Solar Power Rankings Report for the Solar Power Rocks website from 2015 to 2020. The rankings were utilized and referenced by a diverse mix of policymakers, advocacy groups, and media including The Center...
Learn more about Ben Zientara